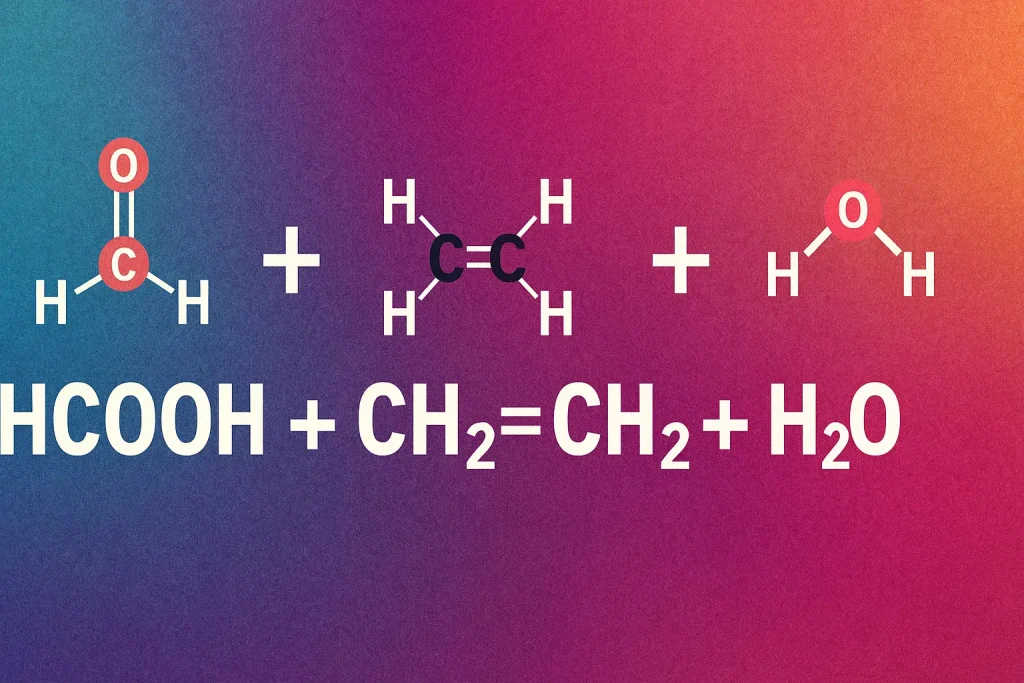
In the vast world of organic chemistry, few combinations are as versatile, intriguing, and impactful as HCOOH (formic acid), CH₂=CH₂ (ethene), and H₂O (water). hcooch ch2 h2o this trio, simple yet powerful, underpins key reactions like acid-catalyzed hydration and esterification, which are foundational to industries, academic research, and green innovation.
Understanding how these compounds interact reveals not only their structural elegance but also their potential for sustainable chemical engineering. From creating esters like ethyl formate to producing biofuel alternatives such as ethanol, these molecules drive reactions that shape our world — quietly but powerfully.
What is HCOOH CH₂=CH₂ H₂O?
The expression “HCOOH CH₂=CH₂ H₂O” refers to a chemical context where formic acid, ethene, and water are present—either as reactants or components of a reaction mechanism. In organic synthesis, their interaction forms a foundation for ester formation, electrophilic additions, and hydration reactions.
This isn’t just textbook chemistry. These reactions reflect key concepts in green chemistry, allowing efficient, low-energy production of valuable compounds like ethyl formate and ethanol. Whether in labs or large-scale industries, this chemical combination serves as a gateway to innovation.
Also Read: https://fizzymag.co.uk/famebloggs-net/
Structural Analysis of HCOOH, CH₂=CH₂, and H₂O Molecules
- Formic Acid (HCOOH): A planar molecule with a carboxylic acid functional group, exhibiting hydrogen bonding and polarity.
- Ethene (CH₂=CH₂): A simple alkene with a double bond between carbon atoms, making it highly reactive in addition reactions.
- Water (H₂O): A polar protic solvent with bent molecular geometry, acting as both a reactant and medium in many reactions.
These structures directly influence reactivity. Formic acid acts as both a Bronsted-Lowry acid and nucleophile. Ethene’s double bond offers a rich site for electrophilic attack. And water, with its hydrogen-bonding capacity, stabilizes intermediates and transition states.
Chemical and Physical Properties That Define Reactivity
Each compound contributes uniquely to chemical behavior. Formic acid, as a weak acid, donates protons readily and forms esters in the presence of alcohols or alkenes. Its boiling point and polarity make it a valuable solvent and catalyst.
Ethene, being nonpolar and unsaturated, shows high reactivity due to its π-bond. It’s a perfect candidate for electrophilic addition reactions, reacting rapidly with acids under the right conditions. Meanwhile, water, though neutral, participates in reactions via protonation, solvation, and hydrolysis, all governed by Le Chatelier’s Principle and thermodynamic equilibrium.
Reaction Mechanisms Between Ethene, Formic Acid, and Water
When CH₂=CH₂ is exposed to formic acid and water, it undergoes fascinating reactions, notably acid-catalyzed hydration and esterification. The mechanisms are anchored in transition state theory, involving protonation, nucleophilic attack, and stabilization of carbocations.
The interplay between kinetics and thermodynamics determines product yield. While formic acid initiates the electrophilic attack, water may stabilize or compete as a nucleophile, depending on concentration, temperature, and catalyst presence.
Electrophilic Addition of Formic Acid to Ethene
This is a textbook example of Markovnikov addition. First, ethene gets protonated, forming a carbocation. Then, formate ion (HCOO⁻) attacks this carbocation, forming ethyl formate.
This esterification with alkenes is efficient, selective, and scalable. Notably, the reaction between ethene and formic acid is mild, making it ideal for green chemistry applications. The reaction’s simplicity belies its massive industrial significance.
Hydration of Ethene in the Presence of Formic Acid as Catalyst
In an alternate pathway, formic acid acts as an acidic catalyst for the hydration of ethene, converting it into ethanol (CH₃CH₂OH). This occurs in polar protic solvents under heat and pressure, aligning with the mechanism of addition reactions.
The result? A clean and efficient production of ethanol, a key biofuel and solvent. The ethene hydration mechanism highlights how even small tweaks in catalyst or temperature shift reaction direction and yield.
Competing Reaction Pathways and Selectivity Control
In a system containing HCOOH, CH₂=CH₂, and H₂O, selectivity is everything. Will it form ethyl formate or ethanol? That depends on temperature, pressure, and catalyst concentration.
Understanding this balance showcases the importance of chemical kinetics vs thermodynamics. The system’s energy landscape defines which reaction pathway dominates. Mastery of this control enables scalable synthesis with minimal by-products.
Formation and Uses of Ethyl Formate in Industrial Chemistry
Ethyl formate (HCOOCH₂CH₃) is a powerful product. It’s used in:
- Fragrance and flavor manufacturing (due to its fruity smell),
- Solvent systems for cellulose and plastics,
- Aerosol propellants.
Its low toxicity, biodegradability, and ease of synthesis make it a sustainable ester in industrial settings. It’s a perfect example of how ester formation reactions support green manufacturing.
Role of Ethanol Formation via Acid-Catalyzed Hydration
Ethanol, produced via the hydration reaction of ethene, is a cornerstone of:
- Biofuel production,
- Pharmaceutical and cosmetic formulation,
- Disinfectants and sanitizers.
This method is a cleaner alternative to fermentation. Its efficiency and reproducibility make it one of the most important industrial uses of ethanol, driving sustainability and reducing carbon emissions.
Green Chemistry Potential of HCOOH, CH₂=CH₂, and H₂O
Each component promotes eco-friendly chemistry:
- Formic acid: Serves as a hydrogen source in fuel cells.
- Ethene: Derived from bioethanol, reducing fossil reliance.
- Water: A green solvent with minimal environmental impact.
Together, they represent green chemistry with formic acid, merging efficiency with sustainability. These reactions demand fewer steps, generate less waste, and offer recyclable options—core principles of the 12 Green Chemistry Principles.
Industrial and Commercial Applications of the Reaction Products
- Ethanol for fuel, cosmetics, and pharmaceuticals
- Ethyl formate in flavors, cleaning products, and coatings
- Formic acid for leather processing, rubber coagulation
- Ethene as a monomer for polyethylene and ethylene oxide
These applications prove the commercial viability and eco-aligned future of these compounds.
Experimental Methods, Conditions, and Safety Considerations
- Reactions typically occur at 60–100°C in glass reactors or pressurized vessels.
- Use of acid-resistant gloves, fume hoods, and eye protection is essential.
- IR and NMR are used to monitor reaction progress and product purity.
- Catalyst concentration and pH control reaction specificity.
Safety and precision go hand in hand when handling reactive organics.
Theoretical Analysis: Thermodynamics and Reaction Kinetics
These reactions sit at the crossroads of thermodynamic equilibrium and activation energy thresholds. Calculating Gibbs free energy, understanding reaction coordinates, and modeling transition states allow chemists to optimize yields.
Advanced methods like DFT (density functional theory) modeling validate the feasibility of desired products under varying conditions, ensuring efficient industrial scale-up.
Spectroscopic Identification of Reaction Products
- Infrared Spectroscopy (IR) identifies functional groups:
- O-H and C=O bands confirm ester or alcohol formation.
- Nuclear Magnetic Resonance (NMR) reveals molecular environment:
- Proton and carbon signals differentiate ethyl formate from ethanol.
These tools provide rapid, non-destructive analysis, key for quality control.
Comparison With Other Carboxylic Acid–Alkene Reactions
- Acetic acid + ethene → ethyl acetate (similar ester pathway)
- Benzoic acid + styrene → bulky ester, less reactive due to sterics
- Methacrylic acid + alkenes used in polymer synthesis
Formic acid’s small size and reactivity make it a uniquely fast and eco-friendly candidate.
Academic and Research Significance in Organic Synthesis
These reactions help teach:
- Reaction mechanisms and regioselectivity,
- Practical application of Markovnikov’s rule,
- Insights into electrophilic vs nucleophilic reactivity.
In research, they inspire new catalysts, biodegradable esters, and low-carbon fuel pathways.
Practical Challenges and Limitations in Lab and Industry
- Water sensitivity can hinder ester yields.
- Volatility of ethene and formic acid demands sealed systems.
- Competing side reactions require fine control of parameters.
Yet, with advances in catalysis and reaction monitoring, these limitations are being steadily overcome.
Environmental Impact and Sustainable Chemistry Insights
These reactions highlight:
- Low carbon footprints,
- Minimal by-products,
- Use of renewable feedstocks.
Perfect examples of green solvents and catalysts enabling real change in industrial processes.
Frequently Asked Questions About These Reactions
Q1: Can formic acid act as both reactant and catalyst?
Yes, in esterification it’s a reactant, and in ethene hydration, it’s a proton source (catalyst).
Q2: Why is ethyl formate eco-friendly?
It’s biodegradable, low-toxicity, and formed in high-yield reactions with minimal waste.
Q3: Is the reaction exothermic or endothermic?
Typically exothermic, especially during electrophilic addition of alkenes.
Q4: What safety precautions are required?
Proper PPE, ventilation, and controlled heating are essential for safe handling.
Final Thoughts
Few reactions showcase the power of simple molecules like the interaction between HCOOH, CH₂=CH₂, and H₂O. Their reactions offer a clean, scalable path to vital chemicals while promoting efficiency, sustainability, and scientific elegance. In a world seeking greener solutions, mastering these fundamental reactions is both a necessity and an opportunity.